The Different Types of Solar Panels
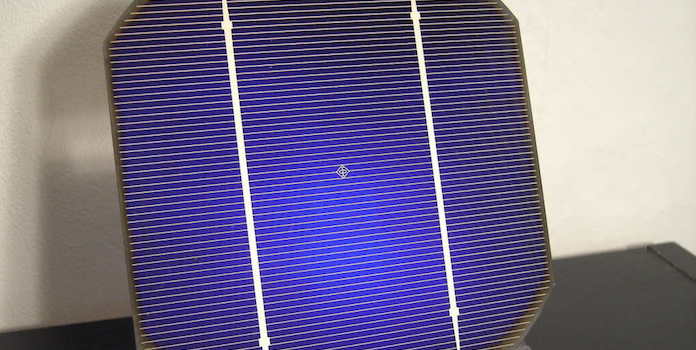
Solar-101: the different types of solar panels.
Solar panels come in many varieties, and there are different types of panels for every occasion. Since going solar is a major investment in your home, it’s good to be in-the-know before you invest. And that’s where we come in.
The Different Types of Solar Panels on the Market
The Greek philosopher Heraclitus said “Change is the only constant in life.” The solar industry is no exception. With the exponential growth of technology and demand for green energy, there are more solar innovations every year.
Despite this, one technology dominates today’s market – crystalline silicon. With hundreds of billions of dollars invested in their manufacturing, R&D, and infrastructure, the market is brutal for newcomers. A bit better won’t cut it. Emerging technologies have to be significantly better in terms of efficiency and cost to warrant a slice of the pie.
But with second-generation thin-film panels floundering, the future now lies in third-generation technologies to break onto the solar scene and change the world energy order as we know it.
We’ll get to them later. First, let’s ogle at the panels taking center-stage on a roof near you:
#1 – Crystalline Silicon Solar Panels (aka the solar panel)
Picture a solar panel… Yeah, that. That’s a crystalline silicon panel. And it commands 92% of the market. When installers say panels, they’re probably talking about these.
The two types of crystalline silicon are:
- Mono-crystalline (mono-Si) – Higher efficiency but is more expensive.
- Multi-crystalline (multi-Si) – Multi-Si provides more Watts for your dollar, so it is more popular. However, making them is incredibly difficult. The silicon must be 99.999% pure, a process that involves 2000 degrees arc-furnaces, complex chemical treatment, and an extremely laborious and energy-intensive procedure known as the Czochralski process, or the multi-Si alternative, the Bridgman process.
Here’s the difference: The Czochralski process produces high-efficiency mono-Si wafers, but at a high cost. The Bridgman process uses less energy and has less material waste so it is cheaper, but the crystals are lower in efficiency. The highest efficiency in the lab for mono-Si modules is 23.8%, but only 19.5% for multi-Si (as of November 2016, see report pg 24). In the real world, the efficiencies are around 17% for mono-Si and 16% for multi-Si (see above report page 26). Thus, mono-Si generates roughly 5% more energy.
What this means for you: Panel efficiency isn’t that important. What’s more important is cost efficiency, i.e. how many dollars are you paying for each Watt of power?
Here’s an example: you can choose system A that’s 5kW and costs $10,000, or system B that’s also 5kW but costs $20,000. System A works at 10% efficiency so the panels are cheaper, and system B is 20%. Which do you choose?
It’s a trick question, because both systems produce the SAME amount of electricity (5kW). They just take up a different amount of space. The one made up of 10% efficient panels takes up twice as much space as the 5kW system made from 20% efficiency panels. If you have infinite space to put them, you choose system A.
So, all things being equal, solar panel efficiency doesn’t matter. Cost efficiency’s the name of the game. Based on 2017 prices, the average $/W cost of multi-Si is $0.364, whereas mono-Si is $0.376/W. Thus, multi-Si is the most cost-effective choice. For a 5kW system, this would be $1880 vs. $1820, so if you choose the less efficient panels, you’re looking at $60 savings.
For our example, with the help of the efficiency rates mentioned above, we know the multi-Si system would take up roughly 10% more space. As we explain in our post on the roof space needed for solar panels, the average rooftop has ample space for a 5kW system, so that shouldn’t be a problem. The concern then becomes installation costs, as installation costs are far more than the panel costs. Also, performance guarantees and product warranties make a big difference. There’s aesthetics to consider, too. SunPower’s panels are more expensive, but they look downright sexy.
#2 – Thin Film (TF) Solar Panels
Meet thin film, the oldest rival to crystalline silicon’s crown, and irrelevant as of 2017.
What they are: A thin layer of photovoltaic material deposited on a substrate, usually glass. Not just thin, but thin thin. A couple of micrometers thin. You’ll mostly find them lounging around in large-scale utility projects, where lack of land isn’t an issue. Why? Despite low efficiency, TFs offered the most Watts for your dollar.
Why they were cheaper: TFs use 1/100th of the photovoltaic material of crystalline silicon. Also, manufacturing is a cake-walk. Put glass into the factory on one side, give the factory a few hours to do its magic, and kazam, a thin film panel out the other side. No fuss, no billion dollar arc-furnaces, no crystal-growing funny business.
Why your green alter ego will love them: TFs have a lower energy payback time (how long it takes to recoup the energy that it costs to make; check out pages 32-33) than mono-Si, and in most cases, multi-Si. CdTe comes in strong: 7 months vs. mono-Si’s 20 months and multi-Si’s 15 months. CIGS is 12 months, and a-Si is 17.
Why They’re Irrelevant: China. A Chinese crystalline panel manufacturing boom, combined with government subsidies, have slashed the price of mono and multi-Si, destroying TF’s price advantage. TF and multi-Si are very similar in price, but the latter is around 16% efficient, and the former only 7-14%.
Emerging Solar Panel Technologies:
#3 – Perovskite Solar Cells
What they are: the love-interest of solar scientists and journalists the world over. Perovskites are a type of crystal, with chemical structure AMX3. A is an organic cation, M is a metal anion, and X is a cation. A subset of these is useful for solar cell applications.
Fun facts: They were first found in the Ural Mountains, and named after the Russian mineralogist, Lev Perovski.
Why they’re so exciting: Take a look at the NREL efficiency chart. Crystalline silicon took almost 20 years to go from 14% to 22% efficiency. Perovskites have taken just 3 years to do the same. Meaning that the efficiency rise of perovskites has been meteoric.
Why they’re poised to revolutionize energy as we know it: For solar energy to become cheaper than fossil fuel alternative, solar panels must be
- high efficiency,
- made of abundant (therefore cheap) materials, or
- simple to manufacture.
(It also helps that they’re not difficult to install as well, as it reduces soft-costs. )
Conventional silicon has one of these: abundant materials. Or maybe one-and-a-half, if you count 24% as high efficiency. Perovskites, on the other hand, are potentially all three:
- First, efficiency.
- Second, abundant materials. Because perovskites are a class of crystal, there are more ways to build a perovskite solar cell than you can think of. The possibilities are endless – organo-metal-trihalide, methylammonium-plumbate-chloride, organo-lead-iodide. Endless possibilities of non-scarce resources? Check. Can you now drop “plumbate” into casual dinner conversation? Check.
- And as for manufacturing, a confused undergrad can spin-coat them in a lab. Energy costs are so low that perovskites have an energy payback time of 2-3 months, more than twice as quick as TF, and up to 10 times that of crystalline silicon. Plus, they could even be combined in tandem with the existing silicon solar infrastructure.
If they’re so great, why aren’t they being used yet? Perovskites have yet to hit the commercial market due to a lack of stability (they degrade in humidity and light). Successful designs so far have used lead, although this could now be a thing of the past, as Oxford University recently found a non-toxic alternative.
#4 – Organic Solar Cells (OSC)
While second-generation thin-film flounders, third-generation thin-film is just getting started. Enter stage left organic solar cells.
Organic does NOT mean: that these are solar cells made from your food-waste and hedge clippings.
Organic DOES mean: that they’re made from organic molecules (molecules made of carbon). The photovoltaic material is almost always a polymer, which is a long chain of (organic) molecules.
Imagine the Scene:
Alessandro: Hey.
You: Hey.
You: That’s a ridiculously stylish suit you’re wearing. At the risk of bowing down to your superior fashion sense, where did you buy it?
Alessandro: Why, it’s the latest solar suit from Armani.
You: Egad?!
(Exit stage left Alessandro).
That’s right folks. Solar clothes, coming to a store near you. And it doesn’t stop there. There’ll be solar tents, solar hammocks, and a myriad of other bizarre places where you’d least expect a solar cell.
Solar clothes? How is this possible?: Two reasons. OSCs are extremely cheap and can be custom designed.
OSCs are cheap because: the organic molecules that make them are mass-produced by the chemical industry, thus way cheaper than your run of the mill silicon feedstock. Also, being a thin-film, their manufacturing is a breeze, aided by the fact that the molecules are solution processable.
OSCs can be custom designed because: the quantity of different organic molecules are only limited by synthetic chemists’ imagination. There are so many possibilities that there’s an organic molecule (if not one in the making) for any situation. A green one for a camouflaged military backpack? Done. A transparent one to put on a window? Done. A tartan-colored one for kilts? Erm… leave it with me.
#5 – Dye-Sensitized Solar Cells
What they are: DSSCs are the (presumably) only solar cell born from spinach. Back in the ’70s, researchers used chlorophyll, a photosynthetic dye, from spinach to show that electricity could be generated from illuminated organic dyes. The next thing you know: Dye-Sensitized Solar Cells. Internally, dye-soaked TiO2 nanoparticles.
Sunlight passes through a transparent electrode and excites electrons within the dye, which flow through the TiO2 and generate a current. They also have high theoretical efficiency, thanks to dyes having low electron recombination and high quantum efficiency. Furthermore, they respond best at low light intensities, absorbing light at higher incident angles better than silicon.
In other words, we’re talking about solar panels, but with a liquid photovoltaic material that has similar theoretical efficiencies than silicon and works best in different light conditions.
What they really, really are: Indoor solar panels.
Why should you be excited: Indoor solar panels. I repeat: indoor solar panels.
#6 – Tandem/Multi-Junction Cells
Your Annual Physics Lesson: Sunlight isn’t made from one type of light. It contains a plethora of different wavelengths: some you can see, like the colors of the rainbow, and some you can’t, like infrared and UV.
A given solar-cell material can only absorb part of the solar spectrum. The maximum that a single material can absorb is 33.7% – the so-called Shockley-Quisser (SQ) limit. Tandem cells get around this by combining two or more photovoltaic materials and thus absorbing multiple parts of the spectrum. This is why tandem cells can achieve far higher efficiencies; the highest two-material cell is at 46%.
Why we’re stuck with lousy 20% Mono-Si panels on the market: In short, money. Tandem cells are complicated to make, resulting in a higher $/Watt than single-material cells. That’s why, historically, tandem cells have only been used in niche applications when space is at an absolute premium, for example, on space rockets.
Why these things will probably take over the world: I say historically, because emerging technologies have thrown the rulebook out the window. Now, it’s possible to create tandem cells by pairing plain vanilla crystalline-silicon with far cheaper photovoltaics, such as perovskites, organics or DSSCs. A collaboration between Arizona State University and Stanford yielded a silicon-perovskite cell with an efficiency of 23.6%, not far off silicon’s all time record of 26.3%
#7 – Quantum Dot Cells
Quantum Dot Cells are another infant technology that’s taking the scientific world by storm.
Why Quantum Dots Are in A League Of Their Own: Remember how single-material solar cells can only absorb a maximum of 33.7% of the solar spectrum? And how tandem cells get around this by combining multiple materials? Quantum dot solar cells, an inorganic semiconductor nanocrystal, have the potential to surpass the Shockley-Quisser limit, no tandem involved.
Quantum dots could surpass the SQ limit by: Multiple Exciton Generation. It’s confusing, but here’s the basic idea: in a conventional solar panel, one light particle (a photon) can only excite one electron, (electrons being the charged particle whose flow produces electric current). In quantum dot cells, absorbed photons can excite multiple electrons. When an electron is excited, you get a corresponding hole as the electron moves from the conduction band to the valence band. The electron-hole pair is called an exciton, hence multi-exciton generation.
If Quantum Dot cells are so great, why are they only 12% efficient on the NREL chart?: Oh, so you’ve read the chart. Well, no-one knows how to control multi-exciton generation yet.
#8: SolarCity Roofing Tiles
What they are: Elon Musk’s taking on of the roofing industry.
What they actually are: A roof made out of some very slick solar panels. Said panels are made from hard-as-steel tempered glass, colored louver film, and a Silevo solar cell.
Why this is genius: For one, there’s no clunky panel-on-roof business. The panels are disguised as normal roof shingles. In fact, from street view, you can’t even tell if it is a solar roof….
Your neighbor: Cool solar roof, bro.
You: What solar roof?
You’ve got options: Tuscan, slate, textured or smooth. And they look absolutely stunning.
In a fight with a 1.2 lb steel ball, the winner is: the solar shingle. It’s “tough as steel”, and “can withstand nearly three times the force of standard roofing tiles.”
Why no-one has tried this before: Actually, it has. As highlighted by the fine folks at Greentech Media, there’s already a long list of companies who have met their untimely integrated-solar demise. As it turns out, it’s a difficult market to break into. Installing such a roof with all its connections is complex, and early-adopters for new roofs can be hard to find.
How much it’ll cost you: Elon Musk & Co have been pretty cryptic with regards to specifications. He said the roof will cost the same as a traditional roof plus the cost of electricity, but that’s about it. However, he compared the solar roof to high-end ceramic and concrete roofing, which cost anywhere in the range of $200-$4000/square (1 square = 100 sq feet), which is significantly higher than your plain-vanilla asphalt at $90/square.
The challenges: Being a roof, there’s no ventilation that normal solar panels enjoy. Thus, these panels will have to be designed to withstand high temperatures which lower efficiency.
When you can get one: Any day now. Tesla-SolarCity is taking orders in April.
Conclusion
As of 2017, the choice for 99% of homeowners is the most basic: crystalline solar panels.
Keep in mind the most important thing here is cost efficiency, performance guarantees, and aesthetics. Of course, emerging technologies could change the game entirely, taking solar from your roof to inside your house or even in your clothes. And when solar goes sartorial, then $/W is probably going to be the least of your concern. But for now, it’s golden.
Image Credit under CC License from Wikimedia – 1, 4, 5, 6, 8; Pixabay – 2; Flickr – 3. Courtesy of Arizona State University – 7